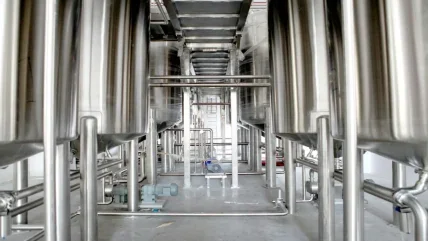
Coal-fired power plants are billion-pound investments that businesses (as well as whole nations themselves) around the world can’t afford to simply discard. However, the combination of old plants, new fuels and new operating conditions leads to corrosion, faults, downtime and inefficiencies for plant operators and manufacturers.
But these kinds of bridging technologies — between the ‘black’ and the ‘green’ — need to be adopted as early as possible to minimise greenhouse emissions from power generation and ensure the sector can meet tightening emissions targets.
Adapting plants to enable them to be co-fired using lower or zero carbon emissions fuels, such as biomass, natural gas or hydrogen, means an urgent need to better understand the forces driving corrosion. High-temperature corrosion is one of the key factors in the degradation of critical components in thermal energy systems. It limits component lives and the efficiencies of thermal energy systems, as well as increasing maintenance costs, risks of failures and the levels of CO2 emissions.
There are several potential types of high-temperature corrosion (such as various forms of hot corrosion and fireside corrosion) that can limit the lives of critical metallic components in thermal energy systems. To counter the range of challenges — and enable the improved selection of materials for critical components that can be more corrosion resistant — a systematic approach has been developed by Cranfield University since 2000 for high-temperature corrosive degradation testing at 300-1100°C. This includes multiple types of corrosion exposures and the scale and location of the damage produced.
Setting a corrosion standard
Establishing a standardised approach to testing is essential for industry. It means the power generation businesses — and the manufacturers of power generation plant — can have more confidence in their selection of materials, and access to more reliable datasets of materials damage distributions under real-life conditions for the purposes of maintenance and capital investment planning. The systematic assessment methods have been, and continue to be, taken up and used by an increasing number of major industrial companies involved with power generation.
At Cranfield the research itself has been focused on exposing materials to specific targeted conditions in controlled atmosphere furnaces, using a ‘deposit recoat’ technique. Assessment of the materials damage has been carried out using dimensional metrology to quantify the damage generated in terms of distributions of metal losses (and any internal damage). This has enabled the research to be scaled up for use on components and probes in pilot and utility-scale plants.
In turn, the methods have facilitated research aimed at improving understanding of the different specific forms of high-temperature corrosion that can be found on materials (alloys and coatings) used in gas turbines and heat exchangers. In particular, the research has contributed to quantifying and understanding the relationships between damage observed in different materials systems and the contaminants in the hot gas stream (from different sources and qualities of fuel and air), gas temperatures and pressures, component surface temperatures and deposits formed on surfaces (in terms of their compositions and fluxes).
Underpinning this approach has been work on investigating and quantifying the relationships between: the component operating conditions (gas temperatures, gas pressures, surface temperatures); the fuel/air compositions used in combustion; and, the component exposure conditions (gas compositions, deposit compositions and deposition fluxes, for example). Changes in these kinds of parameters, such as in deposition flux or in deposit composition arising from differences in fuel compositions (or fuel mixes), can have a significant effect on corrosion damage rates. A further factor that can also influence corrosion damage is the stress state of the components.
Changing fuels
Another strand of research has investigated the potential high-temperature corrosion of candidate materials in future power systems — a UK collaboration coordinated by Cranfield and the US Department of Energy, with participants from industry, laboratories and universities in both the US and the UK.
This has examined how corrosion varies depending on the operating environment, with a view to supporting the operation of gas turbines at higher gas temperatures and with novel fuels. Changing and optimising fuel specifications is one of the routes towards limiting high-temperature corrosion damage in both gas turbines and solid fuel combustion plants.
Among power generation technologies considered are: integrated gasification combined cycle (IGCC) systems, employing biomass/coal derived syngases, or hydrogen enriched syngases, to fire gas turbines; and pulverised fuel combustion systems using advanced ultra-supercritical steam conditions (A-USC) and thus requiring much higher temperature heat exchangers. Assessment of the exposure environments and operating conditions for components in these future systems have been used to guide experimental work and the development of system concepts to optimise the balance between potential efficiencies, fuel specifications and component lives.
These activities have identified materials systems to be used for gas turbine blades/vanes (and materials systems to be avoided) for viable component lives.
For heat exchangers in solid fuel combustion plant, it is possible for specific biomass/coal combinations to generate high damage rates at existing operating temperatures, with a corresponding reduction in heat exchanger lives. We have enabled much better understanding of the factors for assessing the potential for specific biomass/coal combinations to greatly accelerate fireside corrosion damage to superheater or reheater tubes. Quantitative models linking corrosion damage to the most important fuel and operating factors identified have been generated, with industrial partners for their use.
The sheer scale of the value of existing plant, the importance of extending life over replacement, the challenges involved with making adaptations without disrupting operations, means new developments are always likely to be incremental. Innovations are piecemeal rather than revolutionary. But working with the balance between the need to preserve and the need for change, there continues to be progress towards more efficient, lower carbon technologies.
For advanced power generation systems and fuel specification that can act as a bridge to a more sustainable energy infrastructure — the advanced fossil fuel/biomass-fired power systems that are under development — full guidance is being provided for the development of power system concepts to optimise the balance between operating conditions, gas cleaning system requirements, fuel specifications and component lives.
This article first appeared in Modern Power Systems magazine.